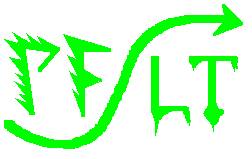
Introduction to Organic Metals
(The lower dimensional, all purpose, solid state, Hubbard Model testing... platform)
Most materials composed of organic molecules are normally not metals because of hybridization, which leaves their conduction and valence bands filled. This property was first overcome by combining planar organic molecules with nonorganic anions (ClO4, PF6 etc.) which serve as acceptors or donors thus resulting in the appearance of partially filled conduction and/or valence bands. Such materials are called charge transfer salts. In 1981 Bechgard synthesized (TMTSF)2ClO4 (see diagram below), the first organic material that was superconducting at ambient pressure. Although, it has a relatively low superconducting transition temperature (1.2 K), the interest in superconductivity and other rather unusual properties in organic materials exploded after this discovery.
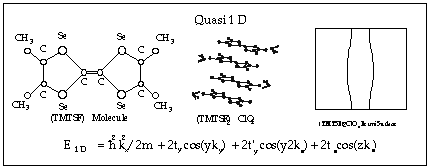
Since 1981, over 700 organic conductors have been synthesized, over 150 of which are superconducting. During this time the superconducting transition temperature in these materials has risen from 1.2 K to 12.6 K (13.2 K under pressure). Also in this time period, a number of other interesting electronic states have been observed in organic conductors including charge density waves (CDW), spin density waves (SDW), field induced spin density waves (FISDW, of course), spin liquids, and the quantum Hall effect. In addition, more traditional quantum oscillation effects that are found in metals, such as the de Haas-van Alphen effect, the Shubnikov-de Haas effect, and angular magnetoresistance oscillations, are greatly enhanced in organic conductors. The study of all the ground state properties is greatly enhanced by the determination of the electronic properties of these materials through the measurement of quantum oscillations. This has caused at least one theoretician to remark that "organic conductors are a laboratory of solid state physics." Some excellent monographs describe the discovery and development of organic superconductors,1, 2 as well as an excellent collection of articles,3 and review articles.4
Because organic conductors are complicated organic salts, they have many free parameters that can be adjusted to carefully fine tune their chemical structure. Consequently their electronic sucture can also be esily adjusted and fine tuned. In addition, their electronic structure is unique because they have low Fermi energies and are electronically very clean, making it easy to study the intricacies of their Fermi surfaces through the observation of quantum oscillations. The low Fermi energy makes high magnetic field experiments more interesting due to the impact of the magnetic energy on the Fermi surface structure. As an example, Ef = 7.0 eV for Pb, whereas Ef for a typical organic is approximately 0.01 eV (50 T ~ 0.003 eV).
So what makes organic conductors such interesting superconductors?
The organic conductors are low dimensional, low carrier density superconductors. That sounds a lot like the high temperature cuprates!
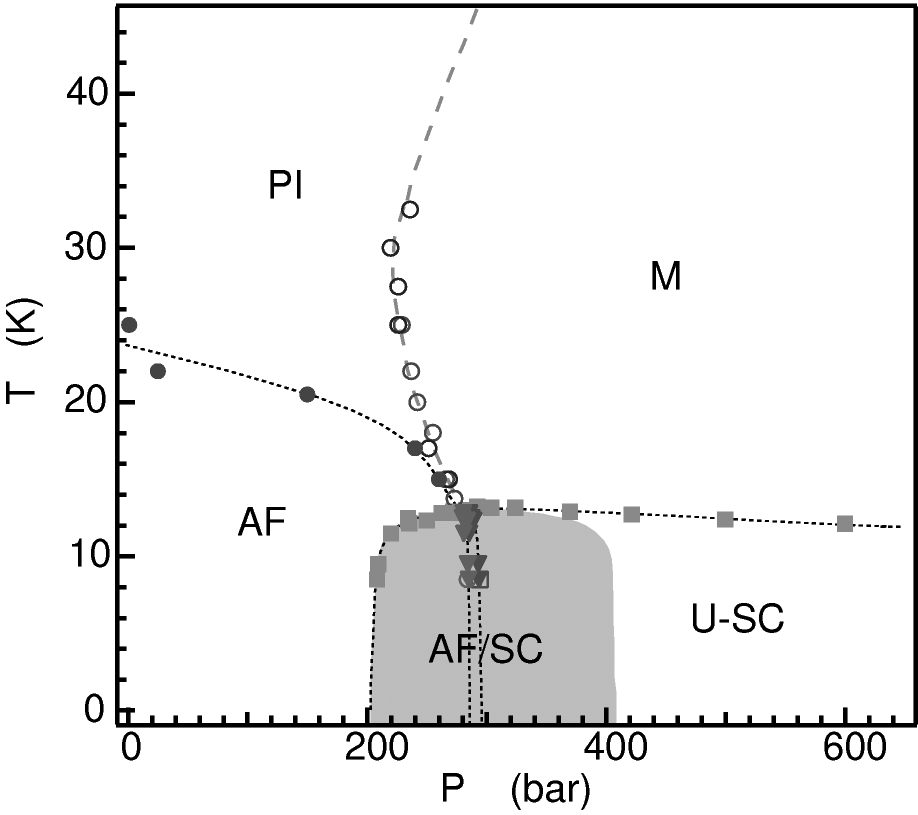
Read on to see the remarkable similarities between families of unconventional superconductors.